Agricultural and specialty crop producers, crop advisors, and extension agents can use this introductory guide on irrigation reservoirs as an overview of irrigation reservoir design basics, water quality and quantity considerations, and routine monitoring and management. Also discussed are funding opportunities through the Environmental Quality Incentives Program (EQIP) for agricultural and specialty crop growers who use or are considering installing an irrigation reservoir(s) as primary or supplemental sources of water for their operations.
In the United States, irrigated agricultural acreage has increased yearly.1 In South Carolina specifically, irrigated acreage has increased from 95,642 acres in 2002 to 210,437 acres in 2017.1 Water for irrigation typically comes from ground or surface sources, including stormwater and recycled water. Competition for ground and surface water sources is increasing. Water availability can be restricted when regions are impacted by drought. Growers can use a reservoir (e.g., tailwater recovery pond or containment pond) to hold captured storm runoff and contain irrigation return water (“operational” water) that have “traveled through production areas and could contain chemical and biological contaminants”.2 Water in irrigation reservoirs is often supplemented by water from municipal sources, pumped from wells, or diverted from other surface waters.
Reservoir Design
Appropriate design of the reservoir is important to meet the water security needs of the farm.3 Additionally, reservoirs should be designed to manage both water and sediment.4,5 The design of irrigation reservoirs is comparable to stormwater detention ponds found in urban areas (figure 1).6 The size of the reservoir varies with the type of operation. Irrigation reservoirs are typically a minimum of 10 ft deep, unless restricted by shallow groundwater depth, and should be large enough to hold the volume of water necessary for the operation for a specific period of time. Historical weather data, including extreme events, can help growers account for water generated during storm events and the frequency of storm events throughout the year when planning for the volume of water to store. Appropriate reservoir design should consider (1) the volume of water entering and leaving the reservoir, (2) the time water resides in the reservoir before exiting or reuse to ensure optimal hydraulic retention time (HRT), and (3) the depth to groundwater. The HRT is defined as the average amount of time that water stays in the reservoir and is estimated by dividing the reservoir volume by inflow rate or outflow rate to the reservoir. In general, HRT quantifies the amount of time it takes for water introduced to the pond to flow out again. Longer HRTs aid in efficient contaminant removal, improving desired quality parameters when recycled for irrigation.7 Increasing HRT is most easily accomplished by increasing the storage capacity of the reservoir and maximizing the distance between where water enters the reservoir (e.g., inlet) and where water leaves the reservoir (e.g., outlet).
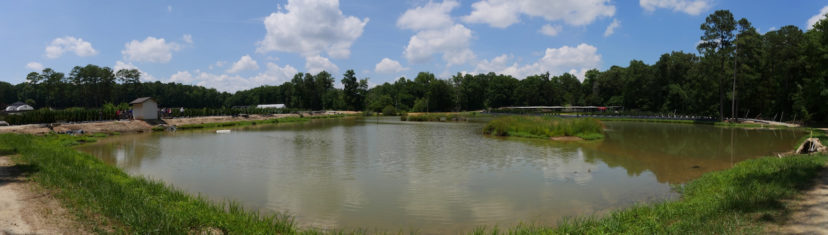
Figure 1. Irrigation reservoir at a plant nursery. Image credit: Sarah A. White, Clemson University.
If installing a new reservoir, consider sizing based on the volume of water needed to irrigate crops during a prolonged drought cycle, when the use of other water sources may be regulated or restricted.8 Develop a drought contingency plan for the reservoir to better meet (and prioritize) water applications during droughts.9,10,11
When determining how to size a reservoir, consider the volume of irrigation return water after an irrigation event,2 current and future water requirements, stormwater runoff during growing and dormant seasons,12 and losses due to evaporation and seepage.6 A reservoir should be sized to contain a minimum of 90% of daily applied irrigation water.3 If an operation irrigates with overhead sprinklers for 1 hour, between 40%-55% of the water applied becomes irrigation return water (i.e., the water that leaves the field following the application of irrigation water).2 Irrigation return rates vary with the type of delivery system: overhead (90%), capillary (48%), and drip (45%).12 The “Reservoir Refill Calculator” calculates how much irrigation water returns to a reservoir after an irrigation event.13
Several other factors, such as the location of the reservoir, distance from the production sites, elevation, slope, groundwater table depth, wildlife, soil type, and proximity to the electrical power supply, should also be considered while designing a reservoir.4,6 For instance, the bottom of a reservoir should be at least 1 m above the groundwater table. Local Natural Resources Conservation Service (NRCS) conservationists can help growers to apply the basic principles and design techniques for reservoirs, and landscape architects can provide additional information and special designs.14 The operator of the farm should consider fencing a reservoir to secure it for safety reasons.
Reservoirs can have multiple inlets, an outlet, and an emergency spillway, in addition to a water withdrawal mechanism (typically a pump). While inlets and outlets help water get into and out of the reservoir, emergency spillways aid in safely conveying excess water without jeopardizing reservoir structural integrity during extreme events that produce extraordinary volumes of water. The inlets can be earthen, gravel, vegetated, plastic-lined, concrete channels, or pipes routing irrigation return water from the production area to the reservoir (figure 2). The slope of the reservoir sides should be chosen to minimize the potential for bank erosion, which could destabilize the reservoir. The slope of the reservoir sides is a function of the soil characteristics, and typically slope can be 3:1 or 4:1 to minimize bank erosion.14,15,16 Additionally, shoreline vegetation could also be selected to minimize bank erosion and better protect reservoir structural integrity.
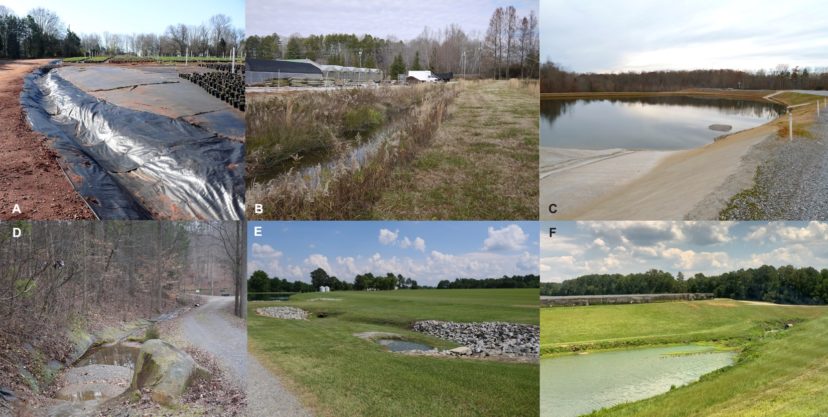
Figure 2. Types of inlets that direct water into irrigation reservoirs (A) plastic-lined, (B) vegetated, (C) concrete, (D) modified pavers, (E) vegetated with riprap for sediment control, and (F) pipe. Image credit: Sarah A. White, Clemson University.
Water Quantity and Quality
Availability of adequate quality water for crop production is becoming more challenging due to the changes in climatic patterns, urbanization, population growth, drought, saltwater intrusion, aquifer depletion, and changes in policies.17 Care and appropriate water conservation efforts must be taken within agricultural operations to extend water supply to meet crop demand. Growers need to develop resilient, on-farm water management practices to remain profitable during water-scarce conditions. Water-resilient management includes implementing efficient irrigation systems and practices, reducing water use, increasing onsite water storage, including retention reservoirs, and identifying an alternate or backup water source.6 Growers will need to invest in infrastructure to recapture, treat, and reuse irrigation return water.18,19,5,20 The primary sources of water for irrigation reservoirs include stormwater and recycled irrigation water (also called irrigation return water, figure 3) supplemented by groundwater, surface water, or municipal reclaimed water.6 Most surface waters are considered “Waters of the United States,” and withdrawals from or releases into them need to comply with South Carolina regulations.21,22 Please refer to the “How the 2020 Definition of WOTUS Affects Agricultural and Specialty Crop Producers” publication for more information on identifying surface waters that constitute Waters of the United States.21 Please refer to “Water Withdrawal Regulations Every Agricultural User in South Carolina Should Know” publication for more information on water withdrawal regulations specific to agricultural water.18
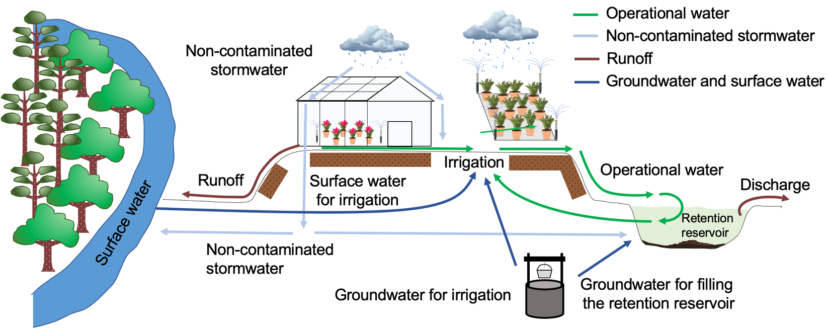
Figure 3. On-farm water classifications. Image credit: Sarah A. White, Clemson University and Mohammad N. Yazdi, The Ohio State University.
Water quality is critical for healthy crop production. Different sources of water within a production area may have different concentrations of agrichemicals, which include nutrients, metals, pesticides, herbicides, insecticides in addition to pathogens, weed seeds, cations, anions (including carbonates), and sediment. Depending on the nature of the operation, stormwater could be considered clean (non-contaminated) or contaminated. For example, water runoff from a greenhouse or building that does not come into contact with production areas is considered clean (non-contaminated). Conversely, water that has been in contact with the crop or production area in which ground has been altered or agrichemicals applied would be considered operational water. Whether generated during a storm or irrigation event, operational water could contain contaminants of concern to growers and negatively impact the surrounding ecosystem including surface waters.5,6 Proper management of operational water plays a pivotal role in controlling the fate and transport of agrichemicals and limiting their escape off-farm.18 The majority of operational water meets recommended irrigation water quality standards and can be reused for irrigation without treatment.23
In some cases, operational water will need to be treated (e.g., acid injection, chlorination, dilution) before reapplication to the target crop or release off-farm to mitigate risk.18 The article by Majsztrik et al.18 provides an in-depth discussion of the various treatment technologies available and the relative costs of each treatment. Some treatment occurs in the reservoir if the residence time (time the water is in the reservoir before its release) in the reservoir before either reusing the water for irrigation or releasing it into the receiving water bodies is adequate (generally, greater than three days). Since many contaminants are sediment-bound, managing sediment movement within the operation also helps to manage water quality.24,25 Water quality can also be improved by designing reservoirs in series to effectively increase the HRT within each reservoir and enhance contaminant (specifically pathogen and nutrient) removal.26 Operators should consider installing various best management practices (BMPs) to minimize erosion and control sediment losses. Vegetated buffer strips and grass swales are generally regarded as inexpensive BMPs that help control sediment prior to the reservoir.27 Forebays, a small settling reservoir or structure in front of the larger reservoir (figure 4), and turbidity curtains (figure 5) are examples of reservoir-related control measures that minimize sediment movement into the reservoir. Other BMPs that reduce the amount of sediment or agrichemical movement into the reservoir include smart irrigation (sensor, plant-based, or weather-based), control release fertilizers, and targeted pesticide applications.
Reservoir Monitoring and Management
Regular reservoir management and monitoring are needed to meet the water demands of an operation in terms of both water quantity and quality. Plan to collect a water sample for water quality analysis at least once a season (spring, summer, and fall) until consistency over time of the water quality parameters is determined.28 For a detailed step-by-step guide on how to collect a water sample, please refer to the “Collecting Samples for Agricultural Irrigation Water Testing” publication. When collecting a sample, collect water away from the bank at the same depth as the irrigation pump intake (ideally near the pump intake) to best characterize the quality of water directly applied to the crops.28 If an extreme (100 + year) storm event occurs, it is wise to test water quality (at minimum – pH and electrical conductivity) to guarantee that desired quality standards are being met.
Various management technologies are available to support water quality reservoir functionality and storage capacity. Inspect the reservoir inlets, outlets, and spillway regularly to ensure structural integrity remains.29 Remove floating debris from the reservoir periodically, particularly after storm events. If aquatic weeds or algae are problematic (clogging pump intakes or safety concerns), consult with your local extension agent or specialist to develop a management plan to mitigate their presence. Aerators can be used to mix the water column (especially in reservoirs more than thirty feet deep) and transfer oxygen throughout the water column while disrupting algal community growth.30 Floating wetlands can be employed to compete with algal communities for nutrients in the water to limit the potential for algal blooms. They may even be useful for limiting plant pathogen survival in water.31 Sediment catch basins or vegetation-based filtration systems at the inlet of the reservoir can reduce sediment and associated contaminants. Periodically (annually at minimum), test the depth of the reservoir to assess sediment accumulation within the structure, especially after an intense storm event using sonar-based monitoring technologies. The depth measurements could help you decide when reservoir dredging is needed to restore storage volume.
If water quality issues are present in the reservoir, various treatment technologies could be deployed to overcome them. Ultra-violet (UV) light can disinfect water containing various bacteria and viruses. Sedimentation tanks could be used to minimize sediment in irrigation water. Flocculants could be used to cluster fine particles and enhance their settling from water. Please refer to Majsztrik et al.18 for detailed information on water treatment technologies, the order of treatment technologies to maximize treatment efficacy, and estimates on cost. For growers who must comply with Food Safety Modernization Act (FSMA) requirements, consult the Food and Drug Administration’s Food Safety Modernization Act webpage for further information guidance on testing and compliance requirements.
Funding Infrastructure Improvements
The Natural Resources Conservation Service (NRCS), as part of the 2018 Farm Bill, has allocated funds through the Environmental Quality Incentives Program (EQIP) to provide “technical and financial assistance to producers to address natural resource concerns and deliver environmental benefits such as improved water and air quality, conserved ground and surface water, increased soil health and reduced soil erosion and sedimentation, improved or created wildlife habitat, and mitigation against drought and increasing weather volatility.”32,33 The technical and monetary assistance through EQIP helps producers voluntarily implement various BMPs. Producers are encouraged to contact their local NRCS office to request assistance and develop a plan to implement BMPs and apply for funding.
Cost-share for all projects is not guaranteed, but technical assistance is. However, “beginning in 2020, States may provide increased payment rates for high-priority practices. In consultations with the State Technical Committee, State Conservationists may designate up to 10 practices to be eligible for increased payments.”32 National and South Carolina priorities related to the installation of a reservoir include reducing non-point source pollution (e.g., sediment, nutrients, pesticides, salinity) and conserving ground and surface water resources to mitigate drought.33 If you are interested in applying to the EQIP, contact your local service center listed on the Service Center Locator website, and visit the USDA NRCS website to start your application.
Conclusions
Irrigation retention reservoirs are critical infrastructure for agricultural producers, especially specialty crop growers. Reservoirs ensure water availability during drought and manage operational water quality. When installing a reservoir, consider designing for the minimum volume capacity you need to enable irrigation during an extended drought scenario – whatever the duration (one week, one month, three months, etc.). When using water from a reservoir, monitor for contaminant presence and determine if the water should be treated prior to use for irrigation. The EQIP through NRCS can provide technical and financial assistance for infrastructure improvements, like reservoirs, but the process requires a time investment to develop a plan in line with NRCS priorities.
Co-Author Partnerships
Support
This material was developed based upon work supported by the USDA-ARS award number 58-5082-0-012.
References Cited
- USDA National Agricultural Statistics Service. 2018 irrigation and water management survey. In: 2017 census of agriculture. Washington (DC): USDA NASS; 2019 [accessed 2021 September 02]. https://www.nass.usda.gov/Publications/AgCensus/2017/Online_Resources/Farm_and_Ranch_Irrigation_Survey/fris.pdf.
- Yazdi MN, Sample DJ, Scott D, Owen JS, Ketabchy M, Alamdari N. Water quality characterization of storm and irrigation runoff from a container nursery. Science of the Total Environment. 2019;667:166–178.
- Ross DS. Containment basin design. In: Green industry knowledge center for water and nutrient management learning modules. Lea-Cox JD, Ross DS, Zhao C, editors. College Park (MD): University of Maryland; 2008.
- USDA Natural Resources Conservation Services. Conservation practice standard: irrigation reservoir. Washington (DC): USDA NRCS; 2002. 436-CPS-1.
- White SA, Owen JS, Majsztrik JC, Oki LR, Fisher PR, Hall CR, Lea-Cox JD, Thomas Fernandez R. Greenhouse and nursery water management characterization and research priorities in the USA. Water (Switzerland). 2019;11:1–19.
- Yazdi MN, Owen JS, Lyson SW, White SA. Specialty crop retention reservoir performance and design considerations to secure quality water and mitigate non-point source runoff. Journal of Cleaner Production. 2021;321:1–16.
- Vymazal J, Březinová T. The use of constructed wetlands for removal of pesticides from agricultural runoff and drainage: a review. Environment International. 2015;75:11–20.
- Fulcher A, LeBude AV, Owen JS, White SA, Beeson RC. The next ten years: Strategic vision of water resources for nursery producers. HortTechnology, 2016;26(2):121–132.
- Lebude AV, Bilderback TE. Managing drought on nursery crops. Raleigh (NC): North Carolina Cooperative Extension Service; 2007. DRO-18.
- Fulcher A, LeBude AV, Owen JS, White SA, Beeson RC. The next ten years: strategic vision of water resources for nursery producers. HortTechnology, 2016;26(2):121–132.
- Lebude AV, Bilderback TE. Managing drought on nursery crops. Raleigh (NC): North Carolina Cooperative Extension Service; 2007.
- Neal CA, Henley RW. Water use and runoff comparisons of greenhouse irrigation systems. Proceedings of the Florida State Horticulture Society. 1992;105:191–194.
- Majsztrik JC, Pitton BJL, Oki LR, White SA. How long will it last? Nursery Management. 2020 January;36(1):6.
- USDA Natural Resources Conservation Service. Ponds: planning, design, construction. Washington (DC): USDA NRCS; 1997. Agricultural Handbook No. 590.
- Virginia Department of Conservation and Recreation. Virginia dcr stormwater design specification no. 14: wet ponds. Version 1.9. Richmond (VA): Virginia DCR; 2011.
- Enciso, J. Small reservoirs for drip irrigation systems. College Station (TX): Texas A&M University; 2018. USDA Grant No. AO172501X443G038.
- Schaible GD, Aillery MP. Challenges for US irrigated agriculture in the face of emerging demands and climate change. In: Competition for water resources. Amsterdam, Netherlands: Elsevier; 2017. p. 44-79.
- Majsztrik JC, Fernandez RT, Fisher PR, Hitchcock DR, Lea-Cox J, Owen JS, Oki LR, White SA. Water use and treatment in container-grown specialty crop production: a review. Water Air & Soil Pollution. 2017;228(4):151.
- Majsztrik JC, Behe B, Hall CR, Ingram DL, Lamm AJ, Warner L, White SA. Social and economic aspects of water use in specialty crop production in the USA: A review. Water (Switzerland). 2019;11:1–13.
- White SA. Wetland technologies for nursery and greenhouse compliance with nutrient regulations. HortScience. 2013;48:1103–1108.
- White SA, Park D, Barrett A, Jones J. How the 2020 definition of WOTUS affects agricultural and specialty crop producers. Clemson (SC): Clemson Cooperative Extension, Land-Grant Press by Clemson Extension; 2020. LGP 1075.
- Barnette E, Sawyer CB, Park D. Water withdrawal regulations every agricultural user in South Carolina should know. Clemson (SC): Clemson Cooperative Extension, Land-Grant Press by Clemson Extension; 2020. LGP 1094.
- Bilderback T, Dole J, Sneed R. Water supply and water quality for nursery and greenhouse crops. Raleigh (NC): North Carolina Cooperative Extension Service; 1999.
- Abdi DE, Fernandez RT. Reducing water and pesticide movement in nursery production. HortTechnology. 2019;29: 730–735.
- Mangiafico SS, Gan J, Wu L, Lu J, Newman JP, Faber B, Merhaut DJ, Evans R. Detention and recycling basins for managing nutrient and pesticide runoff from nurseries. HortScience. 2008;43:393–398.
- Lea-Cox JD, Ross DS. Water management to minimize pathogen movement in containerized production systems. Chapter 32. In: Biology, detection and management of plant pathogens in irrigation water. Hong CH, Moorman GW, Wohanka W, editors. American Phytopathology Society. 2014:377–387.
- Bilderback T, Boyer C, Chappell M, Fain G, Gare D, Gilliam C, Jackson B, Lea-Cox J, LeBude A, Niemiera A. Best management practices: Guide for producing nursery crops. 3rd ed. Atlanta (GA): Southern Nursery Association; 2013.
- Park D, White SA, Hitchcock DR, Younts G. Collecting samples for agricultural irrigation water quality testing. Clemson (SC): Clemson Cooperative Extension, Land-Grant Press by Clemson Extension; 2020. LGP 1084.
- Wallover, GC. Stormwater ponds: Inspection and maintenance considerations. Clemson (SC): Home and Garden Information Center by Clemson Cooperative Extension; 2020. 1881.
- Zhang H, Yan M, Huang T, Huang X, Yang S, Li N, Wang N. Water-lifting aerator reduces algal growth in stratified drinking water reservoir: Novel insights into algal metabolic profiling and engineering applications. Environmental Pollution. 2020;266:1–12
- Bell NL, Garcia Chance LM, White, SA. Clean WateR3: Evaluation of 3 treatment technologies to remove contaminants from recycled production runoff. Acta Horticulturae, 2018. Proceedings of the 3rd International Symposium on Woody Ornamentals of the Temperate Zone; Number 1191:199–205.
- USDA Natural Resources Conservation Service. Environmental quality incentives program. Washington (DC): USDA NRCS; 2019 [accessed 2021 April 07]. https://www.nrcs.usda.gov/wps/PA_NRCSConsumption/download?cid=nrcseprd1469022&ext=pdf.
- USDA Natural Resources Conservation Service. Environmental quality incentives program South Carolina fy, 2021. Columbia (SC): USDA NRCS; 2020 [accessed 2021 April 07]. https://www.nrcs.usda.gov/wps/portal/nrcs/main/sc/programs/financial/eqip/.